For those of you who are interested, I have now made a pdf copy of my thesis available online.
Entitled On the Road to Replacing Oil: A Well-to-Wheels Study Exploring Alternative Transportation Fuels and Vehicle Systems, the study does just that: explores the well-to-wheels energy use and emissions associated with several dozen full well-to-wheels fuel/vehicle combinations.
The study was submitted in June as my undergraduate honors thesis in fulfillment of the requirements of the Robert D. Clark Honors College at the University of Oregon, for which I recieved a 'Pass with Distinction' (the highest marks awarded to undergraduate theses).
The following is the Absract of the study:
Growing concerns about the depletion of world oil supplies and the resulting consequences, as well as concerns about global climate change motivate us to find solutions to replace oil consumption while reducing greenhouse gas emissions. This study explores the performance of various alternative transportation fuels and vehicle systems to reduce consumption of oil and other fossil fuels as well as emissions of greenhouse gases and several harmful pollutants. This study focuses on the light-duty vehicle fleet (all vehicles less than 8,500 pounds) as this segment of the transport fleet accounts for the bulk of transportation oil consumption. To provide a means to objectively compare the energy and emission effects of various fuels and vehicle technologies, this study conducts what is known as a ‘well-to-wheels’ analysis of each of the full fuel/vehicle pathways considered in this study. That is, this study quantifies the energy use and emissions along the entire fuel pathway that are associated with each mile traveled by a vehicle fueled with a specific fuel.To perform the well-to-wheels calculations, I utilized the Greenhouse gases, Regulated Emissions and Energy Use in Transportation (GREET) Model developed by Argonne National Laboratory's Center for Transportation Research. I focused on the year 2025 in order to allow sufficient time for the light duty fleet to change and to allow certain technologies time to develop/reach market (i.e., hydrogen fuel cell vehicles, plug-ins, etc). I performed extensive research to determine appropriate inputs for GREET to represent conditions in 2025 and also modified the GREET model to include several new pathways (and to make other modifications to improve the accuracy and completeness of the model).
Well-to-wheels results were generated for 15 different metrics including:
These results were generated in Btu or grams/vehicle mile travelled.
The following general vehicle and fuel combinations were included in the study:
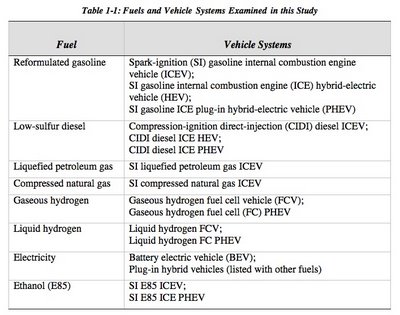
I modeled a total of 70 full well-to-pump fuel pathways (variations of the above general pathways) and 15 different vehicle systems. When combined, these could create over 100 full well-to-wheels fuel/vehicle pathways, but I generate results for only 60 full well-to-wheels pathways (available in an appendix).
The following is the conclusions section of the study (absent footnotes):
The primary motivations for this study were to examine the potential for various alternative transportation fuels and vehicle propulsion systems to reduce petroleum consumption, GHG emissions and criteria pollutants (particularly those occurring in urban areas) resulting from the light-duty transport sector. To do so, this study conducted an analysis of several dozen full well-to-wheels (WtW) fuel/vehicle pathways and presented results using 17 different metrics including total, fossil and petroleum energy use and emissions of three GHGs (as well as total global warming potential-weighted GHGs) and total and urban emissions of five criteria pollutants.Finally, for those of you interested in research in this area, I included a large annotated bibliography that should be useful.
As this study’s results indicate, conducting an analysis of the full WtW pathway for the various fuel/vehicle systems considered is crucial, as upstream, or well-to-pump (WtP), stages contribute significantly to the total energy use and emissions associated with traveling one mile in a given vehicle fueled with a particular fuel. This is true even for criteria pollutant emissions as increasingly stringent vehicle tailpipe emissions regulations (i.e. new Tier 2 and low-sulfur fuel standards) continue to reduce emissions during the vehicle operation, or pump-to-wheels (PtW) stage. Additionally, it is crucial to differentiate between different types of energy inputs as much as possible as energy derived from different sources can have vastly different consequences on resource depletion concerns, energy security and foreign policy. This study thus aimed to provide a more meaningful analysis of the impacts of energy use by dividing energy inputs into total, fossil and petroleum-derived energy. Some studies only present results for total energy inputs. This can be very misleading, as pathways relying largely on renewable energy may appear very costly when total energy is the only metric examined, despite the fact that these pathways may offer considerable fossil and/or petroleum energy use reductions.
This study finds that the fuel production and vehicle operation stages are generally the two most important stages in determining WtW results for the various metrics. The fuel production stage generally has the largest energy losses and results in the most significant emissions of any upstream WtP stage. This is particularly true for petroleum-derived fuels (i.e. gasoline and diesel), ethanol from corn, and especially electricity and hydrogen. Additionally, PtW vehicle fuel economy is particularly important as it directly affects the amount of fuel consumed and thus the level of upstream WtP energy use and emissions associated with traveling one mile in a particular vehicle.
The WtW results generated by this study indicate that there are a variety of alternative fuel/vehicle system pathways that could reduce petroleum consumption related to the light-duty transport sector. All of the alternative pathways considered reduce petroleum energy use somewhat relative to the baseline pathway (i.e., a spark-ignition internal combustion engine vehicle fueled with reformulated gasoline [RFG]), while some nearly eliminate petroleum energy inputs. Alternative (non-PHEV) petroleum-fueled pathways result in petroleum energy use 15-33% less than the baseline pathway, almost entirely due to increased vehicle fuel economy. The pathways that achieve the most significant petroleum energy reductions are those that switch from petroleum to another basic energy feedstock – i.e., natural gas, biomass, or electricity (which is primarily from non-petroleum-fired power plants). Pathways that nearly eliminate petroleum energy inputs include compressed natural gas (CNG) and hydrogen derived from natural gas, as well as all electricity and electrolytic hydrogen pathways. Use of ethanol in E85-fueled vehicles also offers significant petroleum energy reductions of nearly 75% with the remaining petroleum energy use largely due to the fact that E85 contains 15% RFG by volume (and 21% by energy content).
Despite the fact that many pathways result in significant petroleum energy use reductions, several of these pathways (i.e., natural gas and electricity-based pathways) achieve these reductions by substituting other non-renewable fossil fuel-derived energy inputs. In some cases, particularly those reliant on natural gas, this may mean that these pathways offer fewer benefits than indicated by the petroleum use reductions they achieve, as natural gas is also subject to resource depletion and related concerns. Natural gas supplies in North America are already tight and any increased reliance on natural gas for transportation fuels could simply displace concerns about imported oil to concerns about imported natural gas, much of which is located in unstable areas of the world.
This points to the importance of examining fossil energy use in addition to petroleum energy use. The pathways that result in the most significant fossil energy use reductions are clearly those pathways that rely on renewable energy inputs – i.e., the biomass-based ethanol pathways and the electricity-based pathways utilizing energy from remote stranded renewable resources. Several other pathways also result in significant reductions in fossil energy use between 25-65%, however, due to the overall WtW efficiency of these pathways. These include the hydrogen-from-natural gas pathways as well as the battery electric vehicle pathways and most PHEVs fueled with electricity from the U.S. generating mix. Additionally, the ethanol-from-corn pathway results in fossil energy use nearly 35% lower than the baseline due to the use of corn as a feedstock. Thus, there are several pathways that offer significant decreases in both petroleum and fossil energy consumption.
Nearly all of the pathways considered by this study offer some reduction in GHG emissions. GHG reductions are somewhat correlated with fossil energy reductions, although this correlation can be thrown off by the different carbon contents in the various fossil fuels. Natural gas-based pathways, for example, offer larger GHG reductions than fossil energy reductions since natural gas is 21% less carbon intensive (i.e., has 21% less carbon per Btu) than crude oil and 22% less carbon intensive than RFG. In contrast, the GHG reductions for the diesel pathways are slightly smaller than the corresponding fossil energy reductions due to the fact that low-sulfur diesel (LSD) is 6.5% more carbon intensive than RFG.
Still, as with fossil energy reductions, the pathways that offer the most significant reductions in GHG emissions are those that rely on renewable energy inputs, including the biomass-based ethanol pathways and electricity pathways utilizing remote renewables. The remote renewables-based pathways, for example, nearly eliminate GHG emissions, while the biomass-based E85 pathways offer GHG reductions between 72-83%. The remaining GHG emissions for these E85 pathways are the result of combustion of RFG contained in E85 blends.
Several other pathways that rely on feedstocks containing carbon also achieve GHG reductions, however, primarily as a result of high overall WtP efficiencies and/or low vehicle fuel consumption. The hydrogen-from-natural gas pathways and the various PHEV and BEV pathways fueled with electricity from the U.S. generating mix offer reductions on the scale of 40-50% due largely to high PtW efficiencies and zero vehicle operation emissions (or in the case of PHEVs, zero emissions during all-electric driving mode). Additionally, several other pathways, including corn-based E85, CNG, LPG, diesel and the two petroleum-fueled hybrid-electric vehicles (HEVs) offer moderate GHG reductions on the order of 10-35%. In contrast to the biomass-based E85 pathways, emissions for the corn-based E85 pathway are only moderate due to the fact that ethanol plants rely on coal and natural gas for process energy. More intensive use of agricultural chemicals (including nitrogen fertilizers which result in emissions of the potent GHG, N2O) also diminishes the GHG reductions achieved by the corn-based E85 pathway.
Finally, it must be noted that the electrolytic hydrogen pathways are the only alternative pathways that result in increased GHG emissions. In fact, these pathways result in significant increases of approximately 40-80%, despite the fact that hydrogen itself is a carbon-free fuel and hydrogen fuel cell vehicles (FCVs) result in zero emissions of GHGs during vehicle operation. This is due to the GHG-intensive nature of the current coal-dominated U.S. electricity mix and the low WtP efficiency of electrolytic hydrogen production. As such, it may be unwise to adopt the widespread use of the electrolytic hydrogen pathways, as the world cannot afford increased GHG emissions. However, WtW GHG emissions for these pathways are highly dependent on the electricity mix. Thus, electrolytic hydrogen may be acceptable in regions with electricity mixes resulting in low GHG emissions (i.e., California or the Pacific Northwest) and is excellent when specifically utilizing renewable energy (as is evidenced by the remote renewables-to-hydrogen pathways).
This study indicates that, in general, alternative transportation fuels and vehicle propulsion systems help reduce criteria pollutant emissions associated with the light-duty transport sector. In particular, all but one of the alternative pathways results in some decrease in urban emissions of the five criteria pollutants (with the exception being urban NOx emissions from the LSD pathway). However, several pathways result in increased total emissions of one or more criteria pollutants. The electricity-based pathways result in increased PM10 and SOx emissions, for example. Additionally, the ethanol from farmed crops pathways result in increased emissions of NOx and in the case of corn ethanol, a large increase in total PM10 emissions, both due to farming activities.
The criteria pollutant results thus point to the fact that trade-offs may be necessary, as several pathways that perform well in all other metrics result in increases in total emissions of one or more criteria pollutants. This study’s results also point to the importance of upstream stages to WtW criteria pollutant emissions. Thus, in many cases, future efforts to reduce transportation-related criteria pollutant emissions may be forced to focus on WtP stages, rather than on vehicle tailpipe emissions (which have been the focus of increased regulation in the past few decades).
Considering this study’s primary motivations, the WtW results generated by this study provide cause to be optimistic. This study demonstrates that there are several potential alternative vehicle fuels and propulsion systems that can significantly decrease petroleum energy use. The results also indicate that there are several promising options to drastically reduce GHG emissions related to the light-duty transport sector as well as emissions of several criteria pollutants. Care must be taken, however, to avoid simply substituting non-North American natural gas for petroleum use, lest the United States end up embroiled again in the negative consequences arising from reliance on a depleting energy source. Alterative fuels that rely on domestic energy sources should be preferred and renewable resources should be utilized as much as possible.
Some of the technologies and fuels analyzed in this study are ready and available today to contribute immediately to reducing petroleum and fossil energy use and emissions of GHGs and criteria pollutants. Other technologies still have unresolved technological, cost, or other hurdles and may require additional research and financial support to reach the market quickly enough to take full advantage of their potential benefits in the timeframe considered by this study (i.e., by 2025). The results presented in this study can be used as an initial indication as to which technologies should receive the most concerted effort to bring to market. Plug-in hybrid electric vehicles, ethanol derived from woody and herbaceous biomass (i.e., cellulosic ethanol) and electric vehicles all offer a particularly good range of benefits. The pathways utilizing remote stranded renewables offer by far the best benefits, although developing these resources would require large capital investments and considerable planning.
In short, this study finds that the technical options are available to allow significant reductions in petroleum and fossil energy use as well as emissions of GHGs and criteria pollutants related to the light-duty transport sector. What is needed is the development of forward thinking strategies and actions to begin a concerted and rapid transition away from the current oil-addicted light-duty transport sector towards the use of vehicles fueled with energy derived from domestically available and, as much as possible, renewable energy sources. Such vehicles and fuels could also offer dramatically reduced emissions of GHGs and pollutants. This study indicates that the requisite options are available. We must now chart the road forward.
Resources:
7 comments:
Thanks for making this available. I expect it will be very useful as a reference. How hard would it be to add Biodiesel from various sources to the GREET model?
Wow. Your thesis is a very comprehensive and highly technical piece of work. And you did this for an undergraduate degree fulfillment? Impressive. Keep up the good work Watthead.
Tom. I originally did add Biodiesel to the mix (from Soy) and have the figures for that around somewhere. In the end, though, considering how little of our liquid fuels consumption biodiesel from soy can offset, I decided to leave it out to cut the number of pathways I was dealing with down a bit for the final version.
There are SEVERAL pathways I was not able to consider in this study - there's just too darned many of them - including: hydrogen from thermal cracking at high-temp nuclear power plants, hydrogen from gasification of biomass or coal, coal or biomass to liquids (FT diesel or dimethyl ether), and biodiesel from soy, jatropha, or algae (and probably some others that I can't think of right now).
Some of that was because GREET version 1.6 (which I used) was not set up to model those pathways. GREET 1.7 is in public beta stage now and a full version is expected soon. 1.7 will include several of those pathways, for folks interested in researching them.
Oh, and thanks Eric. This was certainly more than I had to do for my undergrad honors requirements - probably the longest and one of the most technical theses submitted this year - but it was what I wanted to set out to do, and I didn't end up half assing it. It ate up far too much of my life for about six months, but it's over now (thank God!) and I have a piece of work I can be happy with.
I'm in the process of working with my thesis advisor to get some version of it published (I'll let you all know when and where if it does get published).
I really would love comments from anyone who actually is interested in reading the beast (as I sometimes call it)...
I've downloaded it and have already read some of it, it's certainly a major piece of work that you must have put an awful lot of work into.
Maybe in my previous posting I didn't put my question terribly well.
Looking at the graph "Selected results: WtW Petroleum Energy Use" say E85 always has some 20% petroleum in it and therefore whenever it's used, we don't get 100% substitution. But that's purely because of the assumption that the gasoline component of E85 is petroleum derived, it could instead be Fischer Tropsch and biomass based gasoline, or it could be gasoline from electrolytic hydrogen from remote renewables and from biomass.
What are your thoughts on this issue?
Heiko, you are correct that the remaining 20% or so of petroleum inputs in all the ethanol pathways is because I assume that the non-ethanol portion of the E85 is petroleum-based reformulated gasoline (as it is in practise). (And remember that the 15% by volume is closer to 20% by energy content as RFG has a higher energy content than ethanol - there's also a bit of petroleum energy inputs from transportation of feedstocks and fuels using petroleum fuels).
As for the possibility of ultimately using a non-petroleum-based fuel as the 15% portion of the E85, that is certainly possible. I didn't model any of the suggested combinations you brought up in your comment, but all are probably possible (I'm not sure about the hydrogen-ethanol mix which might require some retrofitting of the engines).
Those might be worth modeling, but there were just too many possible pathways. In the end, I opted to focus mainly on those pathways that have been suggested as near to mid-term solutions - i.e. ethanol, hydrogen FCVs and PHEVs/EVs for the most part - to present a comparison of these commonly-cited alternative fuels.
There's a ton more out-of-the-box pathways that could be modelled (although they are often harder to get reliable data on since there is less available literature on these obscure pathways). I had originally planned to model solar carbothermic and electrolysis-based zinc-air pathways as well (which would have required the addition of an entirely new pathway to the GREET model), and I may do so in the future, but decided to drop it from my thesis plans.
I did modify GREET to include a couple relatively out-of-the-box hydrogen pathways - i.e., the ones from remote renewables via HVDC or H2 pipeline. These were simple to model though, much easier than say solar carbothermic zinc-air pathway. I am at the point now where I understand the GREET model pretty thoroughly and can modify it pretty freely to do what I want, it just takes some time. I may use it to model some other pathways in the near future; we'll see.
Also, as for as not getting a 100% substitution: I'm not sure that that is necessary, although it is certianly ideal. E85 as the liquid fuel for a Flex fuel PHEV would reduce petroleum demand by about 90%, which would be excellent, in my opinion, and totally doable as well. If our politicians were truly serious about energy independence, they would be working to accelerate the deployment of flex-fuel plug-ins and cellulosic ethanol as soon as possible.
Once we get to 90% petroleum reduction, then we can worry about eliminating the remaining 10% by switching to FT gasoline from biomass instead of the petroleum-based gasoline, or some other option.
Thanks you for your thoughts. I think your reply is fairly summarised as it didn't fit the GREET model / was too complex.
To clear up one minor misunderstanding, when I say hydrogen and biomass, I mean chemically combining the hydrogen with the carbon to get synthetic fuels, not co-firing them.
Thanks for clarifying Heiko. I misunderstood you on the H2 EtOH comment.
Your summary's just about right, although add in 'the fuels you mentioned aren't front-runners deserving immediate attention' and you've probably got it. I tried to focus my attention on a comparison of the immediate front-runners commonly proposed as alternative fuels - there's a lot of hype out there about each option, and I wanted to cut through all that and get down to an actual objective comparison, which I think I accomplished pretty well.
And, of course most of us here already know this, but don't hang your hat on the hydrogen economy! It was one of the least promising of the alternatives I examined - actually, hydrogen via electrolysis at fueling staions using electricity from the grid is by far THE WORST of the alternatives, and actually worse than gasoline in every category except oil consumption!
H2 from electrolysis using remote stranded renewables is fine, although still not as good as using the electricity directly (via HVDC lines to EVs or PHEVs), or even as good as using the H2 only as a transfer medium (an alternative to HVDC) and using it to power a high-temp, high-efficiency stationary fuel cell power plant that co-generates heat/steam and then use the electricity to fuel EVs or PHEVs.
Hydrogen as a transport fuel makes little sense, in my opinion... (other uses, like long distance transportation with on-line storage might be worthwhile, but not as a direct fuel, even if the technical problems can be solved).
Post a Comment